09.05.2018: One Chapter of Nonfiction
- Steven C. Mills
- Sep 6, 2018
- 4 min read
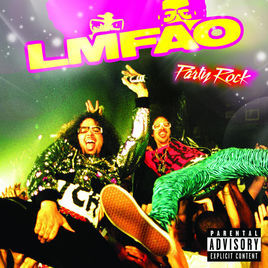
Today's soundtrack is LMFAO: Party Rock, an album that I decided to check out after hearing it referenced on MBMBAM, which I checked out after seeing the acronym referenced on Reddit. My favourite track on the album is definitely "Shots."
This evening, I'm reading the fourth chapter of Stephen Hawking's A Brief History of Time, "The Uncertainty Principle."
Marquis de Laplace determined (heh, a foreshadowing pun!) that since there were so many scientific theories that could be proven, "the universe was completely deterministic" (p. 68). But he took it a step too far. Instead of simply stopping at the reasonable place and saying that "if we knew the positions and speeds of the sun and the planets at one time, then we could use Newton's laws to calculate the state of the Solar System at any other time" (p. 68), which is something that we could all agree with, he went so far as to say that there must likewise be laws that, once discovered, would allow us to predict human behaviours. Some argued that this could not be true, for "it infringed God's freedom" (p. 68); others realized that the belief, though widely held until the early 20th century, could not be true due to the results of several consecutive studies. The first resulted in the calculations of Lord Rayleigh and Sir James Jeans. These two British scientists said that based on the current beliefs, a star could be expected to give out infinite energy, which is impossible. A German scientist named Max Planck decided to address the problem and theorized that energy was not released continuously, but in wave-packets, like how we see in a wave-form of an audio clip. He said that above a certain frequency, the output would require extra energy, meaning that the energy expelled by stars was not infinite, but finite, for "at a high enough frequency the emission of a single quantum would require more energy than was available" (p. 69). It was another German scientist named Werner Heisenberg who put these puzzle pieces together and "formulated his famous uncertainty principle" (p. 69).
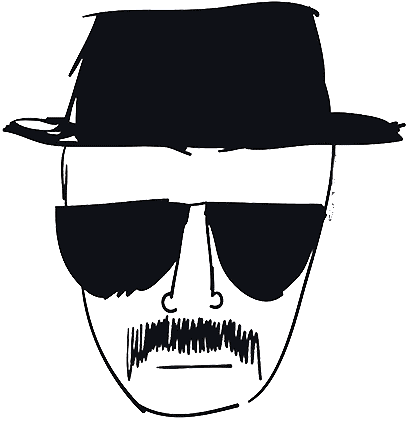
Heisenberg found a paradox. If one wanted to predict "the future position and velocity of a particle" (p. 69), he would first need to accurately its present position and velocity. But to accurately measure a particle's present position and velocity, one would first need to shine a light on it, which would allow the observer to determine its position through its diffusion of the light. However, here arose a problem: the light is emitted in waves, and the position of the particle would not be accurate "between the wave crests of light" (p. 71), necessitating a very short wavelength of "at least one quantum" (p. 71) due to Planck's quantum hypothesis - but the quantum of light "will disturb the particle and change its velocity in a way that cannot be predicted" (p. 71)! The problem boils down like this if we try to measure the position of a particle, we won't be able to judge its speed accurately, and if we manage to judge its speed accurately, we won't be able to judge its position accurately, meaning that measuring the present position and velocity of a particle, the necessary step before predicting a particle's future position and velocity, is impossible.
Laplace had once dreamed of "a theory of science" wherein scientists could predict future events based on current measurements. Heisenberg's Uncertainty Principle shattered this dream. Heisenberg's principle was the foundation of quantum mechanics, a theory that was "based on the uncertainty principle" (p. 72). Quantum mechanics says that particles do not have positions and velocities that can't be measured through observation; instead, they have a "quantum state, which [is] a combination of position and velocity" (p. 73, italics mine). In quantum mechanics, rather than trying to predict a single outcome based on observation, observers determine how many possible outcomes there are, and how likely each one is. Quantum mechanics "introduces an unavoidable element of unpredictability or randomness into science" (p. 73). Albert Einstein disagreed with this, saying that "'God does not play dice'" (p. 73), but he was in the minority. Ironically, Einstein's findings were an integral part of the material that was used in the development of the theory of quantum mechanics. Quantum mechanics "has been an outstandingly successful theory" (p. 73), and it has been successfully applied to nearly every area of science except for "gravity and the large-scale structure of the universe" (p. 73). That area we leave to Einstein's classical general theory of relativity.
Quantum mechanics has given us an understanding of a duality between waves and particles, and their ability to interfere with each other. This understanding has helped us to learn more about "the structure of atoms, the basic units of chemistry and biology and the building blocks out of which we, and everything around us, are made" (p. 78). For example, prior to quantum mechanics, scientists didn't know why atoms didn't collapse in on themselves. Quantum mechanics shows us that electrons orbiting a nucleus are like waves. Their velocities determine their wavelengths. The electrons take every possible path, and those that do not complete a cycle will cancel each other out, leaving only the proper path.
Hawking notes that scientists "do not yet have a complete consistent theory that unifies general relativity and quantum mechanics" (p. 81), but they have identified some features that a successful fusion of the two theories should have, which he says will be discussed further in later chapters.
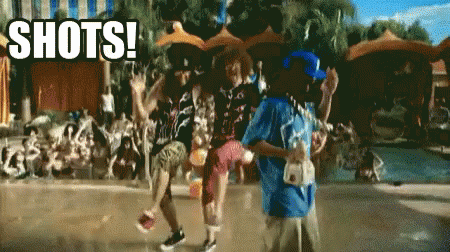
Comments